A survey of blow fly (Diptera: Calliphoridae) populations in Phoenix, Arizona
- 1School of Mathematical and Natural Sciences, New College of Interdisciplinary Arts and Sciences, Arizona State University, Glendale, AZ, United States
- 2School of Life Sciences, Arizona State University, Life Sciences Center, Tempe, AZ, United States
Utilizing insects in legal investigations as a tool for estimating forensically important timelines (e.g., minimum post-mortem interval (min-PMI)) is becoming more commonly used and accepted throughout the world. In the United States much of the climate is temperate, however, the Sonoran Desert is an arid location with extreme heat and irregular rainfall. Work on forensically relevant insects in this region is severely understudied. This study surveyed the populations of forensically important blow flies (Diptera: Calliphoridae) in Phoenix, Arizona for one year using traps baited with four different food sources. Nine species across four genera were collected with Lucilia sericata, Calliphora latifrons, and Lucilia mexicana accounting for 98.6% of total blow flies captured in the baited traps. Abundance drastically changed throughout the year, ranging from 500+ flies to 0 flies captured in a month, with species abundance correlating with temperature and humidity. These results reveal that environmental conditions (i.e., maximum temperature and relative humidity) may limit blow fly activity or seasonally remove (or make inactive) entire local populations, thus affecting their ability to colonize remains and produce an accurate min-PMI.
Introduction
Blow flies (Diptera: Calliphoridae) are among the first insects to arrive to a body after death and use the decomposing remains as a food source for their offspring (Greenberg, 1991; Anderson and VanLaerhoven 1996). Since blow fly larval development stages can be determined via morphological features (e.g., number of spiracles) and is largely temperature dependent (Anderson 2000; Tarone et al., 2011), forensic entomologists can readily determine how far along they are in their development and how long it took them to reach their current stage based on local temperatures (via weather data and developmental data sets). This allows forensic entomologists to use blow flies as biological clocks in death investigations to estimate when the insects colonized the remains known as the time of colonization (TOC), and aid in determining the minimum postmortem interval (min-PMI), which estimates the time that has elapsed since death based on the development of insects feeding on remains (Greenberg, 1991; Byrd and Tomberlin, 2020). While these terms may often be used interchangeably, when insect access to the remains is limited and causes a delay in colonization, the min-PMI needs to be adjusted to account for that delay.
It is well accepted by forensic entomologists that the cold temperatures associated with temperate winters seasonally limit blow fly distributions and activity (Baumgartner, 1993; Cammack and Nelder, 2010; Rosati and VanLaerhoven, 2007). Furthermore, forensic entomologists regularly apply lower base temperature thresholds at which blow fly larval development is assumed to cease (Ames and Turner, 2003). However, no similar assumptions or upper base temperatures exist for blow flies inhabiting hot regions (e.g., deserts), though experiments have shown elevated temperatures reduce blow fly survival, activity, and development (Nicholson, 1934; Byrd and Allen, 2001; Kotzé et al., 2015; Rusch et al., 2019; Rusch et al., 2020). Similarly, other studies investigated the influence of temperature on blow fly oviposition and found 1) temperature induced limits of oviposition (i.e., temperatures at which blow flies stopped laying eggs), 2) temperature specific egg production (i.e., differing numbers of eggs produced across temperatures), and 3) temperature specific timing of oviposition (Ody et al., 2017; Hans et al., 2019; Monzon et al., 2022). Collectively, these studies shed light on how high temperature likely alters the likelihood, timing, and intensity of blow fly colonization. Any delay in colonization is problematic for forensic entomologists as a primary assumption of the min-PMI is that blow flies colonize bodies immediately (i.e., minutes to hours) upon death (Tarone and Sanford, 2017).
The Sonoran Desert environment generally has a very low humidity and can reach temperatures exceeding 40°C during summer months. Information about blow fly presence and distribution in this region is severely lacking. For example, knowledge of calliphorid presence and activity in Arizona is sparse with most relevant studies conducted over 50 years ago (Deonier, 1942; Savage and Schoof, 1955; Schoof and Savage, 1955; Burger, 1965) and unstudied since Baumgartner (1986) reported the arrival of Chrysomya rufifacies (Macquart). While a variety of techniques exist to assess important forensic species in a locale (e.g., pitfall traps, sweep net samples over a carcass, or manual capture of arthropods present at a carcass), bait traps remain a popular option for assessing calliphorid presence because of their low cost and minimal labor requirements. While the effectiveness of small bait traps compared to carrion has not been studied in the Sonoran Desert, studies elsewhere have suggested that small bait traps are largely an effective predictor of early arriving flies to carrion in the same locale (Farinha et al., 2014; Weidner et al., 2017).
A variety of different bait materials have been used to sample calliphorids including fish, cat food, chicken gizzards, and various types of animal liver or muscle (Brundage et al., 2011; Moretti and Godoy, 2013; Farinha et al., 2014; Nakano and Honda, 2015; Weidner et al., 2015), but few studies have investigated which calliphorid species are attracted to which baits. Beef liver, chicken liver, and pork liver are each frequently used in forensic entomological studies but to date no studies have compared the three to determine whether one or a mixture of all three might represent a superior bait trap option. Because blow flies are largely ectothermic, we hypothesize that there will be differences in blow fly diversity and abundance across seasons. Therefore, this study was conducted with the following goals: 1) determine calliphorid species present in Phoenix, Arizona throughout the year, 2) assess how blow fly population numbers correlate with environmental temperatures, and 3) assess calliphorid attraction to four different bait types across seasons.
Methods
Trap design and placement
Four baited traps were placed out weekly for a single 24-hr period across the Arizona State University West campus in Phoenix, Arizona from beginning of June 2019 through the end of May 2020, after which flies were collected, counted, and identified. Trap design followed that of Weidner et al. (2015) and included 60g of a predetermined bait consisting of beef liver, pork liver, chicken liver, or an equal mix (20g:20g:20g) of all three baits. Bait was frozen fresh and then thawed in a refrigerator for approximately 24 hours before the traps were set. The location of each bait was rotated each week to ensure the same bait was not deployed in the same location for multiple weeks in a row. The distance between traps ranged from 332m to 1143m. All traps were deployed between 11am and 1 pm for approximately 24 hours as our focus was initial colonizers of decomposing remains. Upon removal, all adult flies were removed from the trap and frozen at -20°C until identified.
The Arizona State University West campus is located in northwestern Phoenix, Arizona along the border of Glendale, Arizona. The campus is a rectangular parcel of approximately 278 acres. The campus is in a suburban environment, surrounded by residential housing and businesses. Campus buildings are surrounded by desert landscapes and walking paths. Traps were situated near the four corners of campus apart from the northwestern corner of campus, which was inaccessible due to the presence of a large solar array. Site locations consisted of patches of desert trees (either Prosopis veleutina or Parkinsonia spp.) situated at least 250 m from the nearest building in areas where there was enough cover to conceal the traps to decrease chances of disturbances by pedestrians. Traps were suspended approximately four feet off the ground to prevent access by scavengers and were placed among the little-leaved foliage of the desert trees to ensure that each trap was in filtered shade. Traps were placed in similar types of locations to minimize landscape impacts between sites.
Temperature data were collected for the 24-hr period traps were out from the closest station reported (6501 City of GlendaleTemp; ~ 7.71km away) and rainfall data were collected from the closest station reported (Paradise Ln. @ 47th Ave.; 2.81km away), which were obtained from Maricopa County weather single sensor data reports (http://alert.fcd.maricopa.gov/showrpts_mc.html) and reflect the 24 hours that the traps were active.
Species identification
All adult Calliphoridae were identified down to species morphologically, using Jones et al. (2019) excluding two specimens. The two samples were morphologically identified down to the genus Lucilia and confirmed molecularly. Molecular analyses were conducted following the methods of Weidner et al. (2020). After sequencing, barcodes were compared to NCBI data using BLAST. Voucher specimens for each species were deposited into the Hasbrouck Insect Collection (ASUHIC).
Data analysis
To assess changes in blow fly abundances and bait preferences across an entire trapping season, we compared the average number of blow flies captured each month at four sites. Note, while we collected and counted blow flies each week, our analysis uses a resolution of month to allow for each bait type to rotate through each site each month, thus providing an n of 4. Because several of our trapping events resulted in capturing no blow flies, our data were zero-inflated (69.71%). Therefore, we developed a series of generalized linear mixed models (GLMM) to assess their levels of over- and under-dispersion and compared them using Akaike information criterion (AIC) and loglikelihood ratio test (Zuur et al., 2009). Month and meat (i.e., bait type) were modeled as fixed effects, while site was modeled as a random effect. The four models we developed and compared were a GLMM with a Poisson distribution, a negative binomial GLMM, a zero inflated GLMM with a Poisson distribution, and a zero inflated GLMM with a negative binomial distribution. After comparison, the negative binomial GLMM was used for analysis as it had the best fit determined by an AIC score with the lowest value, the greatest loglikelihood, and the second-best dispersion value (see supplemental table 1 for all values and comparisons) of the four models. Note, months 7 and 8 (i.e., July and August) were dropped from the analysis as they had zero blow flies captured and had nothing for comparison purposes. Because month was found to be significant (see supplemental table 2), a posthoc analysis using the package emmeans was used to compare the mean number of blow flies by month (see supplemental table 3). To investigate which climate variables influenced the number of flies, we developed GLMM models containing average weekly trap day (i.e., first 24-hr period trap was set out) relative humidity, and one of three temperature variables (average trap day temperature, maximum trap day temperature, or minimum trap day temperature) as fixed effects, as well as their interactions, again used site as a random effect, and then compared the model fit using AIC (see supplemental table 4). Once the best temperature variable was determined (i.e., model with maximum trap day temperature and RH interaction; see supplemental table 4), we again developed a series of GLMM models as described above due to zero inflation (69.71%) (see supplemental tables 5, 6). All analyses were performed in the software R (version 4.1.3). In addition, we calculated the relative abundance (broken down by month) and species richness for each season and bait type along with a Simpson diversity index (range 0-1; 1-D formula used) for season Seasons were defined by the calendar year and temperature (Table 1) summer (June–August), fall (September–November), winter (December–February) and spring (March–May).
Results
A total of 946 adult calliphorids were collected and identified down to species. These specimens contained eight species spanning four genera (Table 2). The most abundant species was Lucilia sericata (Meigen) (61.0% of all flies collected), followed by Calliphora latifrons Hough (27.5%) and Lucilia mexicana Macquart (10.3%). Of those molecularly tested, several sequences returned as >99.5% match to a series of matching known sequences in the database. Two sequences resulted in 99.8% matches to multiple species (i.e. L. mexicana and Lucilia coeruleiviridis (Macquart)) and therefore cannot be reliably identified using barcoding methods at this time. Lucilia sericata was the most common caught fly in spring (all months but March), summer (no flies captured in July and August), fall and one month in winter (Figure 1). Calliphora latifrons was the most common fly January through March (Figure 1). The species richness was greatest in the spring (8), lowest in the summer (1) and equal (both 3) for fall and winter. When analyzed by month, March had the highest number of species, totaling four (Table 3). When investigating bait type, the species composition of the predominant flies was similar across all traps (Figure 2). However, the species richness was highest in the chicken liver trap (7) and equal across the rest (all 4; Table 4).
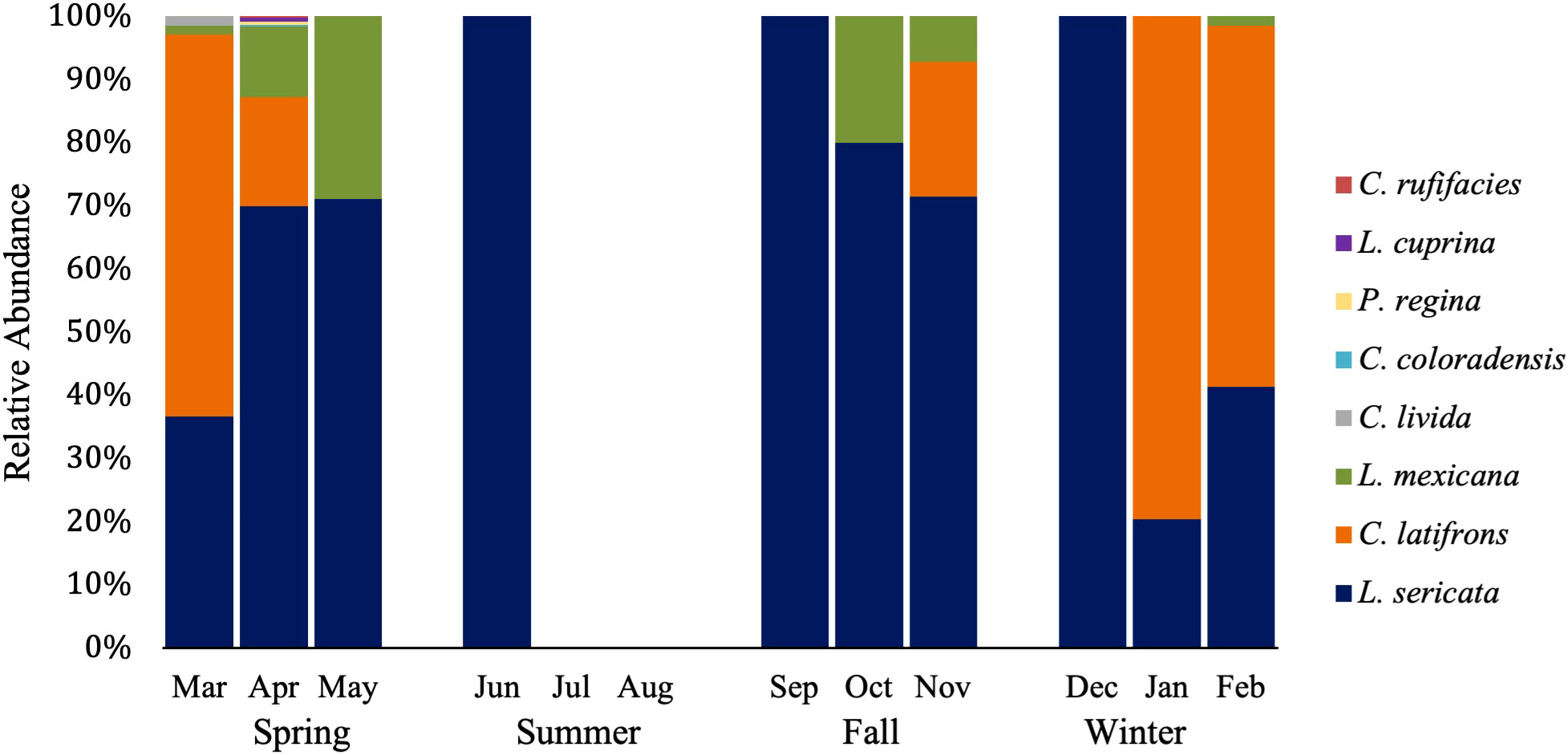
Figure 1 Relative abundance of blow fly species by month. Spring: n=806 (139, 570, 97 respectively), Summer: n=2 (2,0,0 respectively), Fall: n=20 (1,5,14 respectively), and Winter: n=116 (44, 70, 2 respectively).
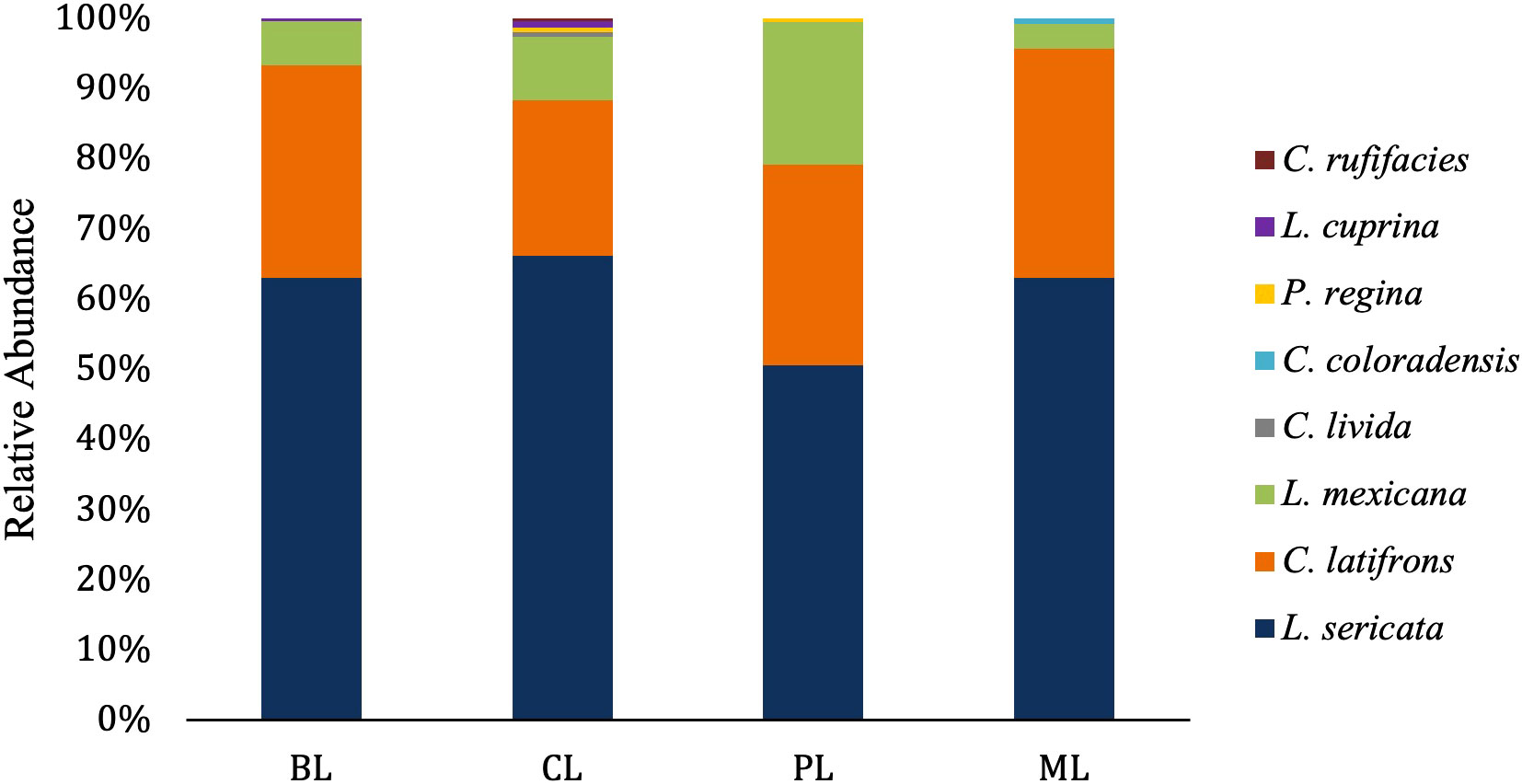
Figure 2 Relative abundance of blow fly species based on bait type. BL: n= 257, CL: n= 311, PL: n=235, and ML: n= 141.
The average number of blow flies differed by month (p < 0.05), but not by bait type (p > 0.05; see supplement Table 2 for a model summary). Average number of blow flies captured was greatest during April and dropped to zero during July and August when temperatures were greatest (>40°C) (Table 1; Figure 3). The most blow flies captured in a single trap was with a maximum trap day temperature of 30˚C (n= 91 flies; Figure 4), an average trap day temperature of 23˚C, and a minimum trap day temperature of 14˚C (month = April; Figures 4–6). Only three traps captured blow flies when the maximum trap day temperature reached 40-41˚C (n=1 and n=42 flies for 41˚C, and n = 1 fly for 40˚C; Figure 4). No blow flies were captured when the maximum trap day temperature reached 42˚C or higher (July and August saw zero blow flies captured with weekly trapping efforts at four separate sites; Figure 4), even when the average and lows still reached 34 and 27˚C. The lowest trap day temperature where blow flies were captured was 4˚C (n=2 flies in one trap one time), though the average and maximum trap day temperatures were 11 and 18˚C. The lowest trap day minimum temperature to capture ten or more blow flies was 10˚C, with average and maximum temperatures of 17 and 25˚C. Similarly, relative humidity was found as a significant predictor of blow fly abundance (see supplemental table 6). Most blow flies were captured when the relative humidity ranged from 14-48%, with the greatest capture event (n=91 flies in one trap) when the relative humidity was 29% (Figure 7). Very few blow flies (n= 0-3 per trap) were captured when the relative humidity was 50% or greater (Table 1). When reviewing the predominant species, L. sericata was captured over a broad range of relative humidity (11-75%) and temperatures (4-41°C). Calliphora latifrons was captured at intermediate to high levels of relative humidity (30-75%) and low to intermediate temperatures (5-32°C). Lastly, L. mexicana was captured at low to intermediate levels of relative humidity (11-46%) and at intermediate to high temperatures (13-41°C) (Supplemental Figures 1A-D).
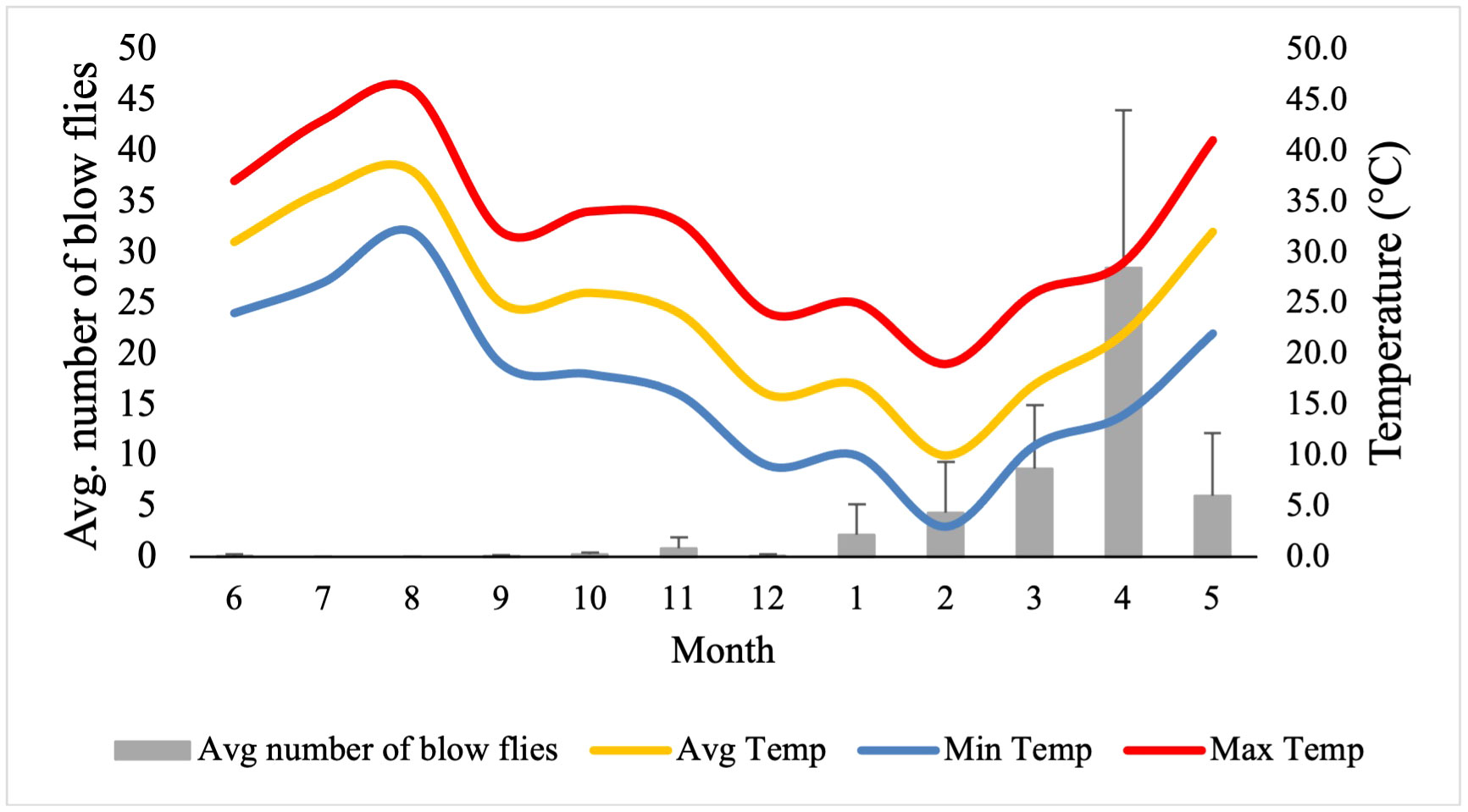
Figure 3 Average number of blow flies captured (grey boxes and left y-axis) with the trap day (24 hour window) average (yellow line), minimum (blue line), and maximum (red line). Error bars are the standard deviaition of each month’s average number of captured blow flies from all four trap sites.
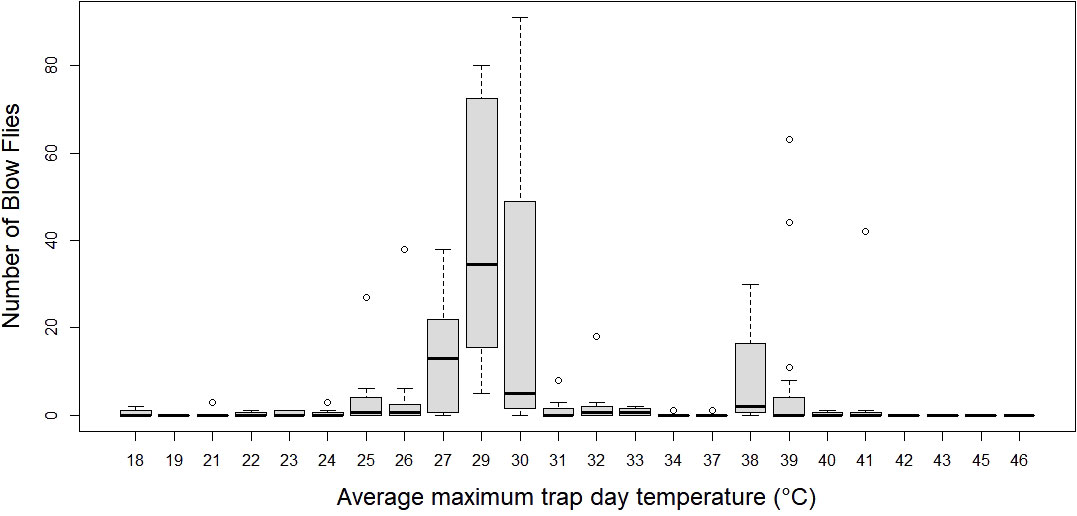
Figure 4 Number of blow flies by average trap day (24 hour window) maximum temperature. Boxplots represent median number of flies with 1.5 x Inter quartile range.
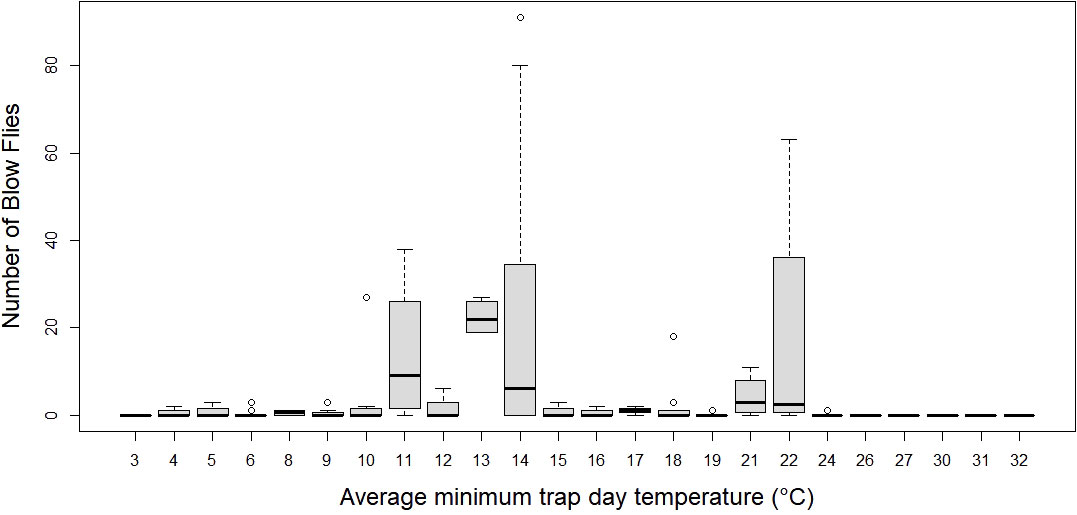
Figure 5 Number of blow flies by trap day (24 hour window) minimum temperature. Boxplots represent median number of flies with 1.5 x Inter quartile range.
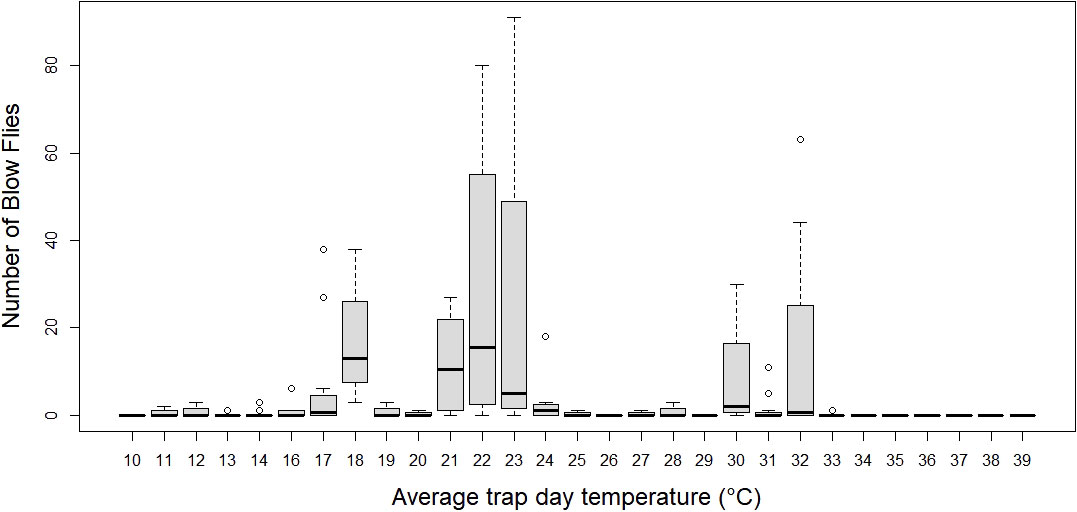
Figure 6 Number of blow flies by average trap day (24 hour window) average temperature. Boxplots represent median number of flies with 1.5 x Inter quartile range.
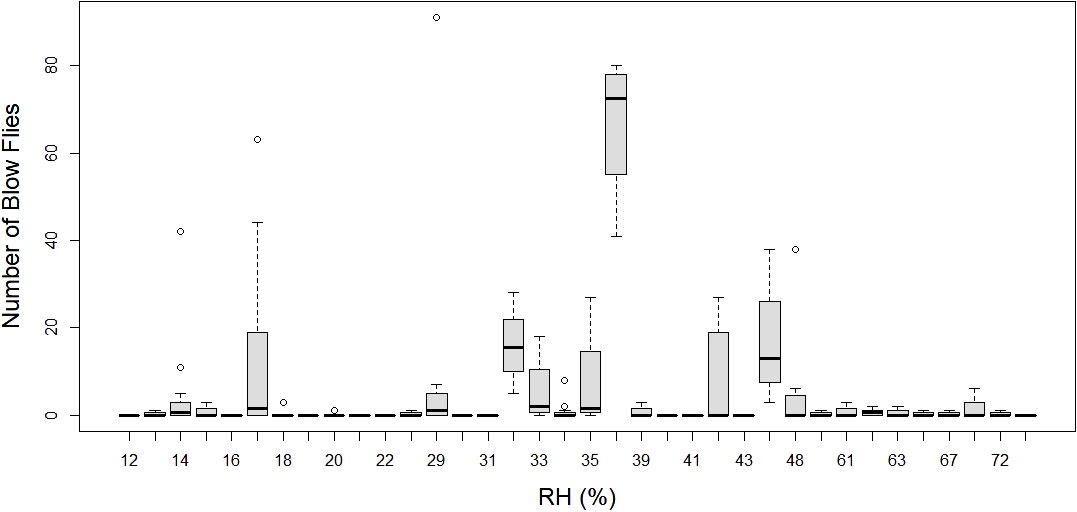
Figure 7 Number of blow flies by average trap day (24 hour window) relative humidity (RH). Boxplots represent median number of flies with 1.5 x Inter quartile range.
Discussion
The biodiversity data presented here are the first survey examining forensically important blow flies in Phoenix, Arizona. Three species, L. sericata, C. latifrons, and L. mexicana, consisted of 98.8% of the calliphorids sampled during this study, with the remaining five species being captured in low numbers. Additionally, the species-level identification of two specimens could not be determined beyond the genus Lucilia morphologically or molecularly in this study. Molecular studies have suggested the existence of two distinct clades for L. mexicana with specimens from Texas and New Mexico indistinguishable from Lucilia coeruleiviridis (Macquart) (DeBry et al., 2013; Williams et al., 2016). These findings also suggest that this is true for at least some specimens in Arizona, but further investigation is warranted. Although no forensically focused survey studies have been conducted in Phoenix, in the 1950’s filth flies were investigated across the United States, including in Phoenix. Although nearly 98% of the sample consisted of L. sericata and Musca domestica Linnaeus, eight forensically relevant blow fly species were detected, including L. sericata, Lucilia cuprina (Wiedemann), L. mexicana, Calliphora coloradensis Hough, C. latifrons, Calliphora grahami, (Aldrich), Cochliomyia macellaria (Fabricus), and Phormia regina (Meigen) with six overlapping in this survey (Savage and Schoof, 1955). Neither C. macellaria nor C. grahami were captured during this study, however Calliphora livida Hall and C. rufifacies were. While L. mexicana was only mentioned as part of the 1.2% of bycatch by Savage and Schoof (1955), it was the third most commonly collected species in our study (10.2% of total collection) and has been confirmed colonizing human remains in the area (personal observation LMW). Two adult C. livida were captured during this study, indicating small populations may be present in the area. Although C. livida has been reported from Arizona in previous survey work (Burger, 1965; Tantawi et al., 2017; Jones et al., 2019), this is the first reported record of the species from Maricopa County. Only a single adult of C. rufifacies was captured during this survey. Chrysyoma rufifacies was first detected in Arizona during the early 1980s (Richard and Ahrens, 1983; Baumgartner, 1986). The larvae of this species display facultative cannibalism and predation. Chrysomya rufifacies often colonizes carrion after other species, so the short deployment time for our bait traps may have largely excluded this and other later arriving species (Weidner et al., 2015). Phormia regina, C. rufifacies, and C. macellaria were observed in the study area during the duration of this experiment (GBIF.org, 2021). Their absence in our trap sample could be due to a variety of factors. In other regions, C. rufifacies has shown an ability to outcompete native carrion flies, including C. macellaria, a species that has historically been common in Arizona (Deonier, 1942; Burger, 1965; Wells and Greenberg, 1992). The activity of C. macellaria in the area may require further study to determine whether its absence in our study was due to trapping methods or a drastic reduction in C. macellaria populations over recent decades.
Another topic that warrants attention is the lack of calliphorid activity observed during July and August, the two warmest months of the survey. In contrast to most forensic entomology studies which see the summer as the height of blow fly activity, data in this study suggests that summers in Phoenix may be too hot for blow fly activity as populations decline when daily highs hit ~35°C and drops to near zero at 40˚C. Temperatures averaged ~32°C and routinely exceeded 40°C when the traps were deployed. Traps were placed around noon, when temperatures were climbing, but still below their peaks, thus allowing for colonization below max daily temperatures. Most blow flies (n=25 flies+ per trap) were captured when maximum trap day temperatures were below 40˚C (only one trapping event caught more than a single blow fly at 41˚C) and minimum trap day temperatures were above 12˚C, giving a range of ~29˚C for regular blow fly activity (e.g., flight and presumed feeding and/or colonization efforts) in Phoenix, Arizona. The results of our field study suggest local blow flies are largely restricted to flight and consequently colonization between temperatures of 13-40˚C. These results align with the flight temperatures observed by Nicholson (1934) (15-40˚C) for four species of blow fly. As with temperature, relative humidity also had a statistical effect on blow fly capture numbers, with the majority of blow flies captured when the relative humidity ranged from 14-48%, and the greatest capture event (n=91 flies in one trap) when the relative humidity was 29%. While maximum trap day temperature and relative humidity (i.e., the variables from the best fit model) did not have a significant interaction effect, they were moderately negatively correlated (correlation = -0.58), revealing that blow fly capture (i.e., a proxy for activity, presence, and consequently colonization potential) was greatest at both moderate temperatures and relative humidity levels. While residents of Phoenix are familiar with extreme temperatures, the frequency and duration of extreme heat events are projected to increase with climate change (Grossman-Clarke et al., 2014). The relationships between blow fly activity and these high heat events are particularly important to understand because these events often cause human mortality through direct means like exposure or by causing larger systematic issues like blackouts. Predicting delays or total absence of colonization in these scenarios may provide an important tool when conducting death investigations in situations involving extreme heat.
The findings from a recent death investigation reported by Wells (2019) described a scene in which a decedent with multiple stab wounds was found in Las Vegas, NV, USA during the summer and presented no carrion insect colonization or activity, even though the body was fully exposed with blood pooled around it. In this case, it was concluded by multiple forensic entomologists that the reason the body had not been colonized was because either the body had not been exposed for long enough for necrophagous insects, such as blow flies, to find it or, that the death happened after sunset and the body was found before sunrise, which could prevent blow flies from finding and colonizing the body as they are largely diurnal. While both explanations are certainly plausible, it is important to recognize that Las Vegas, NV, USA is the #1 urban heat island in the United States (Kenward et al., 2014) with regular summer highs of 40-42°C (NOAA NCEI). The works of Nicholson (1934), Rusch et al (2019; 2020), and Monzon et al. (2022) all found that temperatures ranging between 35-42°C reduced blow fly activity, increased knockdown probability, and decreased oviposition probability, indicating that upper temperatures in hot arid environments, such as Las Vegas or Phoenix summers, may potentially limit or delay blow fly colonization.
Along with duration of exposure, bait type should also be considered when investigating forensically relevant blow fly populations. Brundage et al. (2011) used traps baited with beef liver to assess blow fly diversity and abundance in central California and captured seven calliphorid species. When Nakano and Honda (2015) conducted a follow-up to the Brundage et al. (2011) study in the same area using additional baits, they captured an additional eight calliphorid species. Weidner et al. (2017) compared New Jersey calliphorid species that were present at piglet carcasses with those recovered from traps baited with beef liver and found that the three most frequently collected species were the same between both attractants, although Protophormia terraenovae (Robineau-Desvoidy), which was captured in sweep net samples above the piglet carcasses, was not captured in the liver-baited traps. Moretti and Godoy (2013) baited traps with chicken gizzard, sardines, or beef liver to investigate the preferences of forensically relevant insects in southeastern Brazil and found that all three of the most prevalent calliphorid species preferred chicken, followed by fish. In our study we found no difference in blow fly abundance by bait type but found some variation by species presence. All predominant species were collected at each bait, however, chicken liver collected seven species total, while the remaining traps only captured four.
One concern with bait traps is that the summer heat and arid conditions altered the physical condition of the bait (e.g., cooked or desiccated), making it unattractive to blow flies in the area after a certain time of exposure and depending on the daily environmental conditions. However, a primary assumption in forensic entomology is that blow flies find and colonize remains (i.e., represented by the meat in our traps in this study) almost immediately upon death (e.g., minutes to hours). Thus, nearby blow flies should still have found our traps before the bait was cooked or desiccated as they did in all other (cooler) months. Because zero blow flies were caught in our baited traps for two entire months (with some days cooler than others), we suggest that this may be another “not by the book” scenario (Owings et al., 2022) of delayed blow fly oviposition due to the high temperatures and dry conditions experienced during summer months in Phoenix, Arizona. Such environmental conditions may have been too extreme for blow fly colonization. Because traps were deployed near the heat peak of the day, future Sonoran Desert studies should investigate whether trapping at different parts of the day might yield different results. Mohr and Tomberlin (2014) found time of day to be an influential variable on carcass attendance. While those studies were performed in a more humid, less heated (< 38.2°C) environment, if blow flies in the present study were active during the cooler hours of morning or evening (but not active during the heat peak of the day), the bait may have been dried out and become less attractive by the time they were active.
Conclusion
Decades ago, Deonier (1940) first examined aspects of calliphorid thermal biology, focusing on the minimum temperature requirements for calliphorid activity in Arizona. Today, we consider the importance of examining the other extreme of blow fly thermal biology. Little work preceded Deonier (Nicholson, 1934) or followed (Stavenga et al., 1993; Ngoen-klan et al., 2011; Lutz et al., 2019) and no work assessing blow fly activity due to climatic conditions (e.g., temperature and humidity) has been done in hot, arid, desert environments such as Arizona. However, some laboratory studies have begun examining the upper thermal limits (e.g., oviposition) for populations of adult blow flies in other regions within North America (Ody et al., 2017; Hans et al., 2019; Monzon et al., 2022), including some studies on warm-weather species (e.g., C. rufifacies and C. macellaria) from warm, but humid locations (i.e., Texas, USA); adult flies were exposed to a variety of temperatures for varying lengths of time to assess their survival and “knockdown” (i.e., the point at which an organism can no longer effectively locomote and remain upright) following exposure to elevated temperatures (Rusch et al., 2019; Rusch et al., 2020). Flies of both species show sharp increases in knockdown and sharp decreases in survival when exposed to temperatures above 40˚C, with nearly 100% knockdown and 0% survival when exposed to 45˚C, thus providing a narrow range of temperatures for their upper thermal tolerances. Such findings should be concerning to forensic entomologists when predicting forensically important timelines, such as the time of colonization (TOC) as related to the min-PMI (i.e., time since death). A temperature-induced delay in colonization extends the pre-appearance interval, which is often assumed to be minimal (i.e., minutes to hours) or zero in estimates of the postmortem interval (Wells, 2019; Byrd and Tomberlin, 2020). However, understanding the temperature-colonization relationships should improve estimates of the pre-appearance interval much in the way continued studies of the temperature-development relationships of larval blow flies helps improve estimates of the TOC. Because of climate change, extreme high heat events are occurring and expected to continue to occur with increasing frequency across the desert southwestern United States (Raymond et al., 2022). While the most extreme effects may be seen in the desert, Arizona is a highly diverse state, and temperatures are rising across its deserts, mountains, rivers, and forests. Concurrently, the late summer monsoons that typically bring relief to these regions are often delayed or even entirely absent (McCoy et al., 2022), resulting in increased duration of these extreme temperatures. Blow flies are already the product of extreme specialization as a result of their exploitation of a highly competitive resource, but in the desert southwest, they must also confront temperatures that approach the very limits of their survival. Considering the diversity of the state, the presence of a successful invasive species with a history of displacing competitors in C. rufifacies, and the highly specialized nature of blow fly biology, rigorous study of this region could reveal a wealth of information related to the responses and dynamics of sensitive species to rising global temperatures across much of the southwestern United States.
Data availability statement
The raw data supporting the conclusions of this article will be made available by the authors, without undue reservation.
Ethics statement
The manuscript presents research on animals that do not require ethical approval for their study.
Author contributions
AM and LW contributed to the conception and design of the study. DF, AM, and LW contributed to the data collection and organization. TR performed the statistical analysis. AM, DF, TR, and LW wrote sections of the manuscript. AM, TR, and LW read and revised the manuscript. All authors approved the submitted version.
Acknowledgments
We would like to thank Dr. Terry Whitworth for verifying specimens. We would also like to thank Drs. Jonathan Parrott and Gareth Powell for assistance with the molecular analyses. Lastly, we would like to thank all of the undergraduate researchers who assisted during this research project.
Conflict of interest
The authors declare that the research was conducted in the absence of any commercial or financial relationships that could be construed as a potential conflict of interest.
Publisher’s note
All claims expressed in this article are solely those of the authors and do not necessarily represent those of their affiliated organizations, or those of the publisher, the editors and the reviewers. Any product that may be evaluated in this article, or claim that may be made by its manufacturer, is not guaranteed or endorsed by the publisher.
Author disclaimer
Mention of trade names or commercial products in this publication is solely for the purpose of providing specific information and does not imply recommendation or endorsement by the USDA. The conclusions in this report are those of the authors and do not necessarily represent the views of the USDA. USDA is an equal opportunity provider and employer.
Supplementary material
The Supplementary Material for this article can be found online at: https://www.frontiersin.org/articles/10.3389/fevo.2023.1158347/full#supplementary-material
Supplementary Figure 1 | Total number of blow flies by species and associated climate variable; (A) relative humidity, (B) average minimum temperature, (C) average temperature, and (D) average maximum temperature. Daily temperatures were recorded and averaged over one month while blow fly trapping occurred. Note, x-axes show all values of associated climate variable where blow flies were captured as well as the maximum value recorded while trapping to show full range of recorded values while trapping.
References
Ames C., Turner B. (2003). Low temperature episodes in development of blowflies: implications for postmortem interval estimation. Med. Vet. Entomol. 17 (2), 178–186. doi: 10.1046/j.1365-2915.2003.00421.x
Anderson G. S. (2000). Minimum and maximum developmental rates of some forensically significant Calliphoridae (Diptera). J. Forensic Sci 45(4), 824–832. doi: 10.1520/JFS13964J
Anderson G. S., VanLaerhoven S. L. (1996). Initial studies on insect succession on carrion in southwestern British Columbia. J. Forensic. Sci. 41(4), 617–625. doi: 10.1520/JFS13964J
Baumgartner D. L. (1986). The hairy maggot blow fly Chrysomya rufifacies (Macquart) confirmed in Arizona. J. Entomol. Sci. 21 (2), 130–132. doi: 10.18474/0749-8004-21.2.130
Baumgartner D. L. (1993). Review of Chrysomya rufifacies (Diptera: Calliphoridae). J. Med. Entomol. 30(2), 338–352. doi: 10.1093/jmedent/30.2.338
Brundage A., Bros S., Honda J. Y. (2011). Seasonal and habitat abundance and distribution of some forensically important blow flies (Diptera: Calliphoridae) in Central California. Forensic. Sci. Int. 212 (1-3), 115–120. doi: 10.1016/j.forsciint.2011.05.023
Burger J. F. (1965). Studies on the succession of saprophagous Diptera on mammal carcasses in southern Arizona. Master Thesis (The University of Arizona). Available at: http://hdl.handle/10150/551777.
Byrd J. H., Allen J. C. (2001). The development of the black blow fly, Phormia regina (Meigen). Forensic. Sci. Int. 120, 79–88. doi: 10.1016/j.forsciint.2009.12.007
Byrd J. H., Tomberlin J. K. (2020). Forensic entomology: The utility of arthropods in legal investigations. 3rd ed (Boca Raton, FL: CRC Press).
Cammack J. A., Nelder M. P. (2010). Cool-weather activity of the forensically important hairy maggot blow fly Chrysomya rufifacies (Macquart) (Diptera: Calliphoridae) on carrion in Upstate South Carolina, United States. Forensic. Sci. Int. 195 (1-3), 139–142. doi: 10.1016/j.forsciint.2009.12.007
DeBry R. W., Timm A., Wong E. S., Stamper T., Cookman C., Dahlem G. A. (2013). DNA-based identification of forensically important Lucilia (Diptera: Calliphoridae) in the continental United States. J. Forensic. Sci. 58 (1), 73–78. doi: 10.1111/j.1556-4029.2012.02176.x
Deonier C. C. (1940). Carcass temperatures and their relation to winter blowfly populations and activity in the southwest. J. Econ. Entomol. 33, 166–170. doi: 10.1093/jee/33.1.166
Deonier C. C. (1942). Seasonal abundance and distribution of certain blowflies in southern Arizona and their economic importance. J. Econ. Entomol. 35 (1), 65–70. doi: 10.1093/jee/35.1.65
Farinha A., Dourado C. G., Centeio N., Oliveira A. R., Dias D., Rebelo M. T. (2014). Small bait traps as accurate predictors of dipteran early colonizers in forensic studies. J. Insect Sci. 14 (1), 1–16. doi: 10.1093/jis/14.1.77
Greenberg B. (1991). Flies as forensic indicators. J. Med. Entomol. 28 (5), 565–577. doi: 10.1093/jmedent/28.5.565
Grossman-Clarke S., Schubert S., Clarke T. R., Harlan S. L. (2014). Extreme summer heat in Phoenix, Arizona (USA) under global climate change, (2041-2070). DIE-ERDE–J. Geograph. Soc. Berlin. 145 (1-2), 49–61. doi: 10.12854/erde-145-5
Hans K. R., LeBouthillier R., VanLaerhoven S. L. (2019). Effect of temperature on oviposition behavior and egg load of blow flies (Diptera: Calliphoridae). J. Med. Entomol. 56 (2), 441–447. doi: 10.1093/jme/tjy173
Jones N., Whitworth T., Marshall S. A. (2019). Blow flies of North America: keys to the subfamilies and genera of Calliphoridae, and to the species of the subfamilies Calliphorinae, Lucilinae, and Chrysomyinae. Can. J. Arthropod. Identification. 39. doi: 10.3752/cjai.2019.29
Kenward A., Yawitz D., Sanford T., Wang R. (2014). Summer in the city: hot and getting hotter. Climate Cent. 1, 1–29.
Kotzé Z., Villet M. H., Weldon C. W. (2015). Effect of temperature on development of the blowfly, Lucilia cuprina (Wiedemann) (Diptera: Calliphoridae). Int. J. Legal. Med. 129 (5), 1155–1162. doi: 10.1007/s00414-015-1153-y
Lutz L., Verhoff M. A., Amendt J. (2019). Environmental factors influencing flight activity of forensically impoortant female blow flies in Central Europe. Int. J. Legal. Med. 133, 1267–1278. doi: 10.1007/s00414-018-1967-5
McCoy A. L., Jacobs K. L., Vano J. A., Wilson J. K., Martin S., Pendergrass A. G., et al. (2022). The press and pulse of climate change: extreme events in the Colorado river basin. J. Am. Water Resour. Assoc. 58 (6), 1076–1097. doi: 10.1111/1752-1688.13021
Mohr R. M., Tomberlin J. K. (2014). Environmental factors affecting early carcass attendance by four species of blow flies (Diptera: Calliphoridae) in Texas. J. Med. Entomol. 51 (3), 702–708. doi: 10.1603/ME13149
Monzon M. A., Weidner L. M., Rusch T. W., Nehrozoglu S., Hamilton G. (2022). High Temperature Limits of Survival and Oviposition of Phormia regina (Meigen) and Lucilia sericata (Meigen). Insects 13 (11), 991. doi: 10.3390/insects13110991
Moretti T. D. C., Godoy W. A. C. (2013). Spatio-temporal dynamics and preference for type of bait in necrophagous insects, particularly native and introduced blow flies (Diptera: Calliphoridae). J. Med. Entomol. 50 (2), 415–424. doi: 10.1603/ME12187
Nakano A., Honda J. (2015). Use of DNA sequences to identify forensically important fly species and their distribution in the coastal region of Central California. Forensic. Sci. Int. 253, 1–13. doi: 10.1016/j.forsciint.2015.05.001
Ngoen-klan R., Moophayak K., Klong-klaew T., Irvine K. N., Sukontason K. L., Prangkio C., et al. (2011). Do climatic and physical factors affect populations of the blow fly Chrysomya megacephala and house fly Musca domestica? Parasitol. Res. 109, 1279–1292. doi: 10.1007/s00436-011-2372-x
Nicholson A. J. (1934). The influence of temperature on the activity of sheep-blowflies. Bull. Entomol. Res. 25 (1), 85–99. doi: 10.1017/S0007485300012529
NOAA NCEI (n.d) Climate at a Glance: Statewide Time Series, Nevada. National Oceanic and Atmospheric Administration (Asheville, NC: National Centers for Environmental Information). Available at: https://www.ncdc.noaa.gov/cag/statewide/time-series/26/ (Accessed January 12, 2023).
Ody H., Bulling M. T., Barnes K. M. (2017). Effects of environmental temperature on oviposition behavior in three blow fly species of forensic importance. Forensic. Sci. Int. 275, 138–143. doi: 10.1016/j.forsciint.2017.03.001
Owings C. G., McKee-Zech H. S., Schwing S. T., Bugajski K. N., Davis M. C. (2022). Not by the book: Observations of delayed oviposition and re-colonization of human remains by blow flies. Insects 13, 879. doi: 10.3390/insects13100879
Raymond C., Waliser D., Guan B., Lee H., Loikith P., Massoud E., et al. (2022). Regional and elevational patterns of extreme heat stress change in the US. Environ. Res. Lett. 17 (6), 064046. doi: 10.1088/1748-9326/ac7343
Richard R. D., Ahrens E. H. (1983). New distribution record for the recently introduced blow fly Chrysomya rufifacies (Macquart) in North America. Southwestern. Entomol. 8 (3), 216–218.
Rosati J. Y., VanLaerhoven S. L. (2007). New record of Chrysomya rufifacies (Diptera: Calliphoridae) in Canada: predicted range expansion and potential effects on native species. Can. Entomol. 139 (5), 670–677. doi: 10.4039/n06-061
Rusch T. W., Adutwumwaah A., Beebe L. E., Tomberlin J. K., Tarone A. M. (2019). The upper thermal tolerance of the secondary screwworm, Cochliomyia macellaria Fabricius (Diptera: Calliphoridae). J. Thermal. Biol. 85, 102405. doi: 10.1016/j.jtherbio.2019.102405
Rusch T. W., Faris A. M., Beebe L. E., Tomberlin J. K., Tarone A. M. (2020). The upper thermal tolerance for a Texas population of the hairy maggot blow fly Chrysomya rufifacies Macquart (Diptera: Calliphoridae). Ecol. Entomol. 45 (5), 1146–1157. doi: 10.1111/een.12902
Savage E. P., Schoof H. F. (1955). The species composition of fly populations at several types of problem sites in urban areas. Ann. Entomol. Soc. America 48 (4), 251–257. doi: 10.1093/aesa/48.4.251
Schoof H. F., Savage E. P. (1955). Comparative studies of urban fly populations in Arizona, Kansas, Michigan, New York, and West Virginia. Ann. Entomol. Soc. America 48 (1-2), 1–12. doi: 10.1093/aesa/48.1-2.1
Stavenga D. G., Schwering P. B. W., Tinbergen J. (1993). A three-compartment model describing temperature changes in tethered flying blowflies. J. Exp. Biol. 185, 325–333. doi: 10.1242/jeb.185.1.325
Tantawi T. I., Whitworth T. L., Sinclair B. J. (2017). Revision of the nearctic calliphora robineau-desvoidy (Diptera: calliphoridae). Zootaxa 4226 (3), 301–347.
Tarone G. S., Picard G. S., Spiegelman G. S., Foran G. S. (2011). Population and temperature effects on Lucilia sericata (Diptera: Calliphoridae) body size and minimum development time. J. Med. Entomol. 48(5), 1062–1068. doi: 10.1603/ME11004
Tarone A. M., Sanford M. R. (2017). Is PMI the hypothesis or the null hypothesis? J. Med. Entomol. 54 (5), 1109–1115. doi: 10.1093/jme/tjx119
Weidner L. M., Gemmellaro M. D., Tomberlin J. K., Hamilton G. C. (2017). Evaluation of bait traps as a means to predict initial blow fly (Diptera: Calliphoridae) communities associated with decomposing swine remains in New Jersey, USA. Forensic. Sci. Int. 278, 95–100. doi: 10.1016/j.forsciint.2017.06.014
Weidner L. M., Jennings D. E., Tomberlin J. K., Hamilton G. C. (2015). Seasonal and geographic variation in biodiversity of forensically important blow flies (Diptera: Calliphoridae) in New Jersey, USA. J. Med. Entomol. 52 (5), 937–946. doi: 10.1093/jme/tjv104
Weidner L. M., Nigoghosian G., Yoho K. C., Parrott J. J., Hans. K. R. (2020). An analysis of forensically important insects associated with human remains in Indiana, USA. J. Forensic. Sci. 65 (6), 2036–2041. doi: 10.1111/1556-4029.14531
Wells J. D., Greenberg B. (1992). Interaction between Chrysomya rufifacies and Cochliomyia macellaria (Diptera: Calliphoridae): The possible consequences of an invasion. Bull. Entomol. Res. 82 (1), 133–137. doi: 10.1017/S0007485300051543
Wells J. D. (2019). A forensic entomological analysis can yield an estimate of postmortem interval, and not just a minimum postmortem interval: an explanation and illustration using a case. J. Forensic. Sci. 64(2), 634–637. doi: 10.1111/1556-4029.13912
Williams K. A., Lamb J., Villet M. H. (2016). Phylogenetic radiation of the greenbottle flies (Diptera, Calliphoridae, Lucilinae). Zookeys 568), 59–86. doi: 10.3897/zookeys.568.6696
Keywords: blow flies, Calliphoridae, Sonoran Desert, forensic entomology, survey
Citation: Meeds AW, Rusch TW, Falcone DL and Weidner LM (2023) A survey of blow fly (Diptera: Calliphoridae) populations in Phoenix, Arizona. Front. Ecol. Evol. 11:1158347. doi: 10.3389/fevo.2023.1158347
Received: 03 February 2023; Accepted: 29 August 2023;
Published: 21 September 2023.
Edited by:
M. Eric Benbow, Michigan State University, United StatesReviewed by:
María Pérez-Marcos, Instituto Murciano de Investigación y Desarrollo Agrario y Alimentario (IMIDA), SpainDavid Rivers, Loyola University Maryland, United States
Copyright © 2023 Meeds, Rusch, Falcone and Weidner. This is an open-access article distributed under the terms of the Creative Commons Attribution License (CC BY). The use, distribution or reproduction in other forums is permitted, provided the original author(s) and the copyright owner(s) are credited and that the original publication in this journal is cited, in accordance with accepted academic practice. No use, distribution or reproduction is permitted which does not comply with these terms.
*Correspondence: Lauren M. Weidner, lauren.weidner@asu.edu
†Present address: Travis W. Rusch, United States Department of Agriculture. Arthropod-borne Animal Disease Research Unit, Agricultural Research Service, Manhattan KS, United States